Neutrino Physics and Astrophysics
Ed Kearns, James Stone, Lawrence Sulak
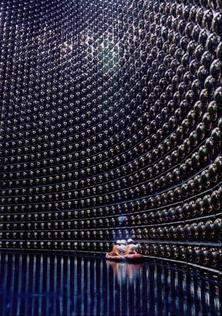
As a sequel to the combined effort of the two earlier deep underground ring-imaging detectors, IMB and Kamiokande, Super-Kamiokande started recording data deep inside a lead and zinc mine in the Japanese Alps in 1996. The detector is a 40-meter high by 40-meter diameter stainless steel tank, filled with 50,000 tons of ultra-pure water. The walls of the tank are lined with 11,200 photomultiplier tubes, each an enormous 50 centimeters in diameter. One half of the surface of the tank is covered by photosensitive material. These tubes record the Cherenkov light from charged particles as they pass through the water.
Physicists in the US and in Japan designed Super-K to search for, in part, the radioactive decay of the proton, a rare event never before observed. Detecting proton decay would confirm the Grand Unified Theory of particle physics.
Though Super-K has yet to detected a single candidate, it observed something equally intriguing: convincing evidence that neutrinos have mass. Because neutrinos carry no charge, they rarely interact with other particles. Billions go through each of us every second without effect. But since Super-K is so large, it detects a handful of these neutrinos each week. Using the data collected by Super-K, researchers including Boston University physicists Jim Stone (US co-spokesman), Larry Sulak and Ed Kearns confirmed results from the two precursor experiments that about half the neutrinos expected were unseen.
Neutrinos come in three species – the tau neutrino, the muon neutrino, and the electron neutrino. Super-K discovered that these neutrino species transform into each other. If oscillations occur, muon neutrinos could transform into tau neutrinos, and the missing neutrinos observed by Super-K would be explained. This was documented in the 1999 Ph.D. thesis of Boston University graduate student Mark Messier. Quantum mechanically, for these oscillations to occur, the neutrino must have mass. Several experiments since this revelation in 1998 have reinforced this interpretation.
The discovery of massive neutrinos has forced theorists to rethink the Standard Model of particle physics; neutrino mass is not anticipated in this most accurate and predictive of theories. Further, with mass, the neutrinos in the universe account for nearly as much mass as all the stars. Hence, they would influence the formation of galaxies in the early universe. This discovery is the first indication of new physics beyond the Standard Model.