
Lawrence R. Sulak
Office Hours: Monday 9 am - 7 pm, any time eLab is open, and by appointment or by phone anytime.
Research Interests:
Unification of the Particles & Forces of Nature using Novel Calorimetric Detectors
My main focus is exploring the unification of particle physics using novel calorimetric detectors. With colleagues, I designed and built the first massive liquid scintillator calorimeter and large area drift chambers, and performed the analysis that discovered neutral weak currents using neutrino beams in 1974. This work was the first to demonstrate that two of the four forces, the weak and the electromagnetic, are unified.
I then designed and prototyped a Cherenkov detector to search for the predicted signal of Grand Unification (proton decay) and for neutrino oscillations, which we discovered.
Applying the Cherenkov detection techniques to quartz fibers, my collaborators and I developed the forward calorimeter for the CMS detector at the Large Hadron Collider. This was instrumental in the discovery of the Higgs boson.
I love and nurture my students. So that they could be formed in the most challenging of world laboratories, I established in 2009 the BU/CERN/University of Geneva Program, the only one in the world to send junior physics majors (15) each year for a 6 to 8-month CERN internship.
Selected Publications:
For a full list of publications, please select the "View CV" tab at the right of the photo above.For downloads of the selected talks below, please see my research website :
"Early history of the discovery of neutrino oscillations", colloquium at the celebration of the 2015 Nobel Award, Uppsala, Sweden.
"La naissance de l'astronomie du neutrino et son avenir," Keynote, 25th Anniversary, CPPM Lab, University of Marseille.
"The Birth of Neutrino Astrophysics," Colloquium, McGill University.
"From the Big Bang to the End of Time," All University Lecture, Carnegie Mellon University.
"Grand Unification: the Demise of the Proton," Collins Lecture, Massachusetts General Hospital Endowed Series.
"From Alchemy to Quarks," Lectures in the Boston University Core Curriculum by LRS.
"The BU Internship Program at CERN for Junior Physics Majors," the only physics study abroad program at CERN.
Education:
- Ph.D. in Physics, Princeton University
- M.A. in Physics, Princeton University
- B.S. in Physics, Carnegie Mellon University
Biography:
Lawrence R. Sulak studied CP violation under Wolfenstein (Carnegie Mellon, BS ‘66), Fitch (Princeton, PhD ‘70), and Turlay (at Saclay and CERN ‘71).
At Harvard, building the first two large (~100 T) totally absorptive calorimeters for FNAL and then BNL, he, Rubbia and collaborators at Penn and Wisconsin discovered weak neutral currents contemporaneously with CERN. Sulak’s team was the first to observe ν-nucleon elastic scattering (1975), further evidence for a unified electroweak force.
To search for proton decay, supernova ν’s, and ν oscillations, Sulak and his students at Harvard proposed (‘78) the first massive (10,000 T) water Cherenkov ring-imaging calorimeter. He, colleagues from Michigan, and collaborators from Irvine built that detector (IMB), proving (‘83) that the nucleon lives > 1032 years. However, they observed an anomalously low number of atmospheric νµ’s, that could not be confirmed by the competing Japanese experiment until 1986 after adopting several of the IMB technical strategies.
IMB was the first to observe neutrinos from a gravitational collapse (‘87) concurrently with the Japanese Kamiokande detector, confirming the models of supernova implosion and setting many limits on the properties of neutrinos.
Later, IMB collaborators and the Japanese united to build a combined second-generation detector, Super-K. They verified that both atmospheric and accelerator ν’s oscillate, proving that neutrinos have mass, a first indication of physics beyond the Standard Model.
To search for point sources of TeV neutrinos, Sulak and collaborators in France built the Antares Observatory, 2.4 km deep in the Mediterranean off the coast of France.
Applying Cherenkov technology to quartz fibers, Sulak spearheaded the development of the CMS Forward Hadronic Calorimeter, contributing to the discovery of the Higgs boson.
Modeled after his ‘78 proposal for IMB, 10 massive ring-imaging calorimeters in 7 countries on 4 continents have now studied neutrinos from accelerators, cosmic rays, reactors, and our sun.
Sulak’s honors include the 2018 Panosfsky Prize, the 2017 APS Instrumentation Award, the Rossi Prize of the American Astronomical Society, the Marseille Research Prize, the Asahi Prize, Fellowship in the American Physical Society, and a Guggenheim Fellowship.
Honors/Awards:
Panofsky Prize, American Physical Society, the top APS award for an experimental physicist (2017)
Instrumentation Award, American Physical Society, Division of Particles and Fields (2017)
Physics Breakthrough Prize in Fundamental Physics, shared with SNO and collaborators in Super-K (2015)
Most Distinguished Alumnus Award, Carnegie Mellon University (2006)
ASAHI Prize, “Discovery of the Finite Mass of Neutrinos,” shared with Super-K collaborators (1998)
Bruno Rossi Prize, American Astronomical Society for "Discovery of Neutrinos from Supernova 1987a" shared with IMB collaboration (1989)
Fellow, American Physical Society (1984)
In the news:
- Super-K and T2K awarded Breakthrough Prize in Fundamental Physics
- Neutrino Nobel has BU connections
- CERN experiments observe particle consistent with long-sought Higgs boson
- First Long-Baseline Neutrino in T2K
- The Large Hadron Collider: it's live
Research Descriptions:
Higgs Detection at The Compact Muon Solenoid Detector at the Large Hadron Collider

The Compact Muon Solenoid (CMS) is a 14 kiloton detector designed to search for new physics at an unprecedented distance scale of 10-19 m at the CERN Large Hadron Collider (LHC). The detector consists of 220 square meters of silicon pixels and strips (80 million channels) for precision charged particle tracking, 75k lead-tungsten crystals for precision electron and photon measurements, a highly segmented 1000-ton brass hadron-calorimeter plus a quartz-fiber forward calorimeter to measure jets from quark and gluon scattering and energy balance, all surrounded by precision muon chambers embedded in the return yoke of the magnet. Overall, the detector features nearly complete solid-angle coverage and can precisely measure electrons, photons, muons, jets and missing energy over a large range of particle energies. These broad capabilities of the CMS detector allow the exploration of electroweak symmetry breaking and will enable the potential discovery of physics beyond the Standard Model.
The CMS trigger and data acquisition systems are responsible for ensuring that physics-enriched data samples with potentially interesting events are recorded with high efficiency and good quality. The experiment has a two-level trigger system, unlike most other hadron collider experiments that have more traditional three-level systems. The first physical level is hardware-based and is called the “Level-1 Trigger” (L1) while the second physical level is software-based and is called the “High-Level Trigger” (HLT). L1 uses information from the calorimeters and muon detectors and is designed to select, in less than 1 ms, the most interesting events starting from a total input (collision) rate of about 40 MHz. The HLT processor farm further decreases the event rate from around 100 kHz to around 400 Hz, before data storage. The trigger system, therefore, has to provide a high selectivity of ~10-5 with respect to the active LHC bunch crossings while ensuring that the ability to select rare, exotic events is preserved. Boston University has played a leading role in the coordination of the CMS Trigger effort with Bose serving as the CMS Trigger Coordinator (2014-2016) and as the CMS Deputy Trigger Coordinator (2011-2013). Group members were involved in the design and commissioning of the CMS HLT and continued to spearhead the effor thereaftert. Many of the BU personnel resident at CERN served as HLT on-call experts during the LHC Run 1 (Avetisyan, Carerra, Fantasia and Sperka) and Run 2 (Avetisyan, Rankin, Richardson).
The collision rate at the LHC also presents enormous technical challenges on the design of readout electronics due to the intense radiation environment and the high speed at which millions of channels of data must be processed. The Boston University group has a leadership role in calorimeter electronics and related software (Rohlf and Sulak). The group has designed and built the data concentrator, a sophisticated piece of digital electronics based on modern field programmable gate arrays (FPGAs) to read out the hadron calorimeter. Group members have also led the custom design of the 18-channel hybrid photo-diode used to convert scintillation light from the calorimeter into electrical signals. We have also designed the electronics to feed calorimeter signals into the muon trigger to greatly reduce the backgrounds in the online event selection. Members of the BU CMS team have also helped design the two hadronic forward calorimeters which are positioned at either end of the CMS detector, to pick up the myriad particles coming out of the collision region at shallow angles relative to the beam line. Group members also play a key role in the day-to-day operation of the calorimeter.
The Boston University collaborators (Bose, Rohlf, and Sulak, their postdocs Girgis and Pinna, and their grad students, with their engineers Eric Hazen and Shouxiang Wu) are reaping the physics benefits of our investment in detector hardware, trigger and algorithm development. Group members are making significant contributions to physics analyses involving searches for new particles and the hunt for the Higgs Boson. These analyses include (among many others) searches for heavy gauge bosons and exotic WZ resonances. We are also playing a leading role in the study of diboson production; the latter is critical for increasing the sensitivity of our Higgs searches and thereby help understand the mechanism of electroweak symmetry breaking.
Bose has served as the co-convener of the Electroweak Diboson group (2011), co-convener of the Resonances group within the Beyond Two Generations physics group (2012-2014), and co-convener of the Beyond Two Generations group (2016-2017). She is currently serving a two-year term as CMS Physics Co-coordinator (2017-2019). Sulak serves on the Advisory Board of the Hadron Calorimeter, and manages some 15 undergraduates as Director of the BU/CERN/DOE Internship Program.
Neutrino Physics and Astrophysics
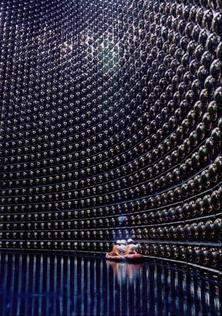
As a sequel to the combined effort of the two earlier deep underground ring-imaging detectors, IMB and Kamiokande, Super-Kamiokande started recording data deep inside a lead and zinc mine in the Japanese Alps in 1996. The detector is a 40-meter high by 40-meter diameter stainless steel tank, filled with 50,000 tons of ultra-pure water. The walls of the tank are lined with 11,200 photomultiplier tubes, each an enormous 50 centimeters in diameter. One half of the surface of the tank is covered by photosensitive material. These tubes record the Cherenkov light from charged particles as they pass through the water.
Physicists in the US and in Japan designed Super-K to search for, in part, the radioactive decay of the proton, a rare event never before observed. Detecting proton decay would confirm the Grand Unified Theory of particle physics.
Though Super-K has yet to detected a single candidate, it observed something equally intriguing: convincing evidence that neutrinos have mass. Because neutrinos carry no charge, they rarely interact with other particles. Billions go through each of us every second without effect. But since Super-K is so large, it detects a handful of these neutrinos each week. Using the data collected by Super-K, researchers including Boston University physicists Jim Stone (US co-spokesman), Larry Sulak and Ed Kearns confirmed results from the two precursor experiments that about half the neutrinos expected were unseen.
Neutrinos come in three species – the tau neutrino, the muon neutrino, and the electron neutrino. Super-K discovered that these neutrino species transform into each other. If oscillations occur, muon neutrinos could transform into tau neutrinos, and the missing neutrinos observed by Super-K would be explained. This was documented in the 1999 Ph.D. thesis of Boston University graduate student Mark Messier. Quantum mechanically, for these oscillations to occur, the neutrino must have mass. Several experiments since this revelation in 1998 have reinforced this interpretation.
The discovery of massive neutrinos has forced theorists to rethink the Standard Model of particle physics; neutrino mass is not anticipated in this most accurate and predictive of theories. Further, with mass, the neutrinos in the universe account for nearly as much mass as all the stars. Hence, they would influence the formation of galaxies in the early universe. This discovery is the first indication of new physics beyond the Standard Model.
The MACRO Experiment: Monopole Astrophysics and Cosmic Ray Observatory
The deep underground MACRO detector operated at the Laboratori Nazionali del Gran Sasso in Abruzzo, Italy from 1990 to 2000. MACRO had a geometrical acceptance of 10,000 square meters at an average depth of 3.8 kilometers of water equivalent under the mountainous overburden of the Gran Sasso d'Italia. The MACRO detector was used to research several topics. The specialty of MACRO was the search for magnetic monopoles: particles with bare north or south magnetic charge. These particles are a natural consequence of Grand Unified Theories, which also predict that the monopole will be very massive, perhaps 10 to the power 16 GeV. Such particles can only be produced by the intense energies available during the big bang. MACRO operated like a giant Time-Of-Flight counter to detect the unique signature of a slow moving but penetrating massive particle. It was equipped with tanks of liquid scintillator, planes of streamer tubes and plates of track etch material in the hopes of recording a convincing signature from a single candidate event. MACRO's high-resolution tracking and timing were also used to perform high statistics measurements of cosmic ray muons; in particular, the scintillator timing was used to distinguish upward going muons produced by neutrino interactions in the rock. This was an opportunity to investigate the possible flavor oscillation of massive neutrinos as suggested by the atmospheric neutrino puzzle, and MACRO was the first experiment to independently confirm the atmospheric neutrino oscillation signal seen by Super-Kamiokande.